Dark matter is like the Rome of astronomy, all observations lead to dark matter. The problem is that physicists and astronomers, don't know what it actually
is. The observations which support dark matter come from many different independent observations, so it is not just some observational error. The observations which corroborate the dark matter paradigm make for a fantastic discussion, but for right now I would like to focus on explanations for what dark matter may be. Specifically, what kind of particles are dark matter?
Dark Matter is not like any particle before
The most attractive candidate theory for dark matter would be simple, it would be motivated from fundamental particle physics, and it would make testable predictions for future observations. Whatever dark matter particles are we know that dark matter does not interact with photons, is electrically neutral, is highly non-relativistic, and it is dissipationless; so basically that means dark matter is boring. To drive at what dark matter really is I would first ask, can even begin to understand the observations without tying ourselves to any specific models for dark matter's nature? I do not know. I am merely going to outline the fundamentals of a plausible dark matter model and address how it confronts observations. There are dark matter particles theories invoked from supersymmtery, universal extra dimensions, and branes; the most common dark matter candidate which I will focus on here is the so called weakly interacting massive particle (WIMP). If dark matter is a WIMP, then we still have only narrowed down what kind of particle it is to a zoo of particles. If we want a pure motivation for an unknown dark matter particle we could go back to the early 1930's when Enrico Fermi developed a theory of beta decay that involved the neutrino and necessitated a new mass scale in nature; this mass scale introduces new fundamental particles that would interact weakly with regular matter and presto, WIMPs. It turns out that WIMPs, particularly supersymmetric neutralinos, are a compelling fit given the current data, but as more precises observations accumulate the theory faces scrutiny. There was a plague of rumors of an affirmative dark matter detection a year ago which were of course unfounded (it took much restraint not to throw in my lot with the speculations). Today dark matter looms as a unsolved problem because its existence is not in question, only its origin.
A plausible theory for dark matter
Dark matter particles zoom right through matter without interacting, similarly to neutrinos, but unlike neutrinos dark matter particles are very massive. The gravitational potential of the largest structures in the universe, like galaxies and galaxy clusters, is dominated by dark matter. Dark matter is everywhere (yes, even here on Earth dark matter is present and it does have some gravitational effect, but it is infinitely more feeble compared to the Earth or the Sun), but dark matter doesn't clump, it doesn't form dark molecules, and it doesn't form dark galaxies. Regular matter that you or I are made of (known as baryonic matter) is gravitationally attracted to dark matter (non-baryonic matter), but dark matter dominates because there is so much of it. It is hard to notice dark matter because it only interacts with regular matter through gravity for the most part and gravity is the weakest of forces. Dark matter remains like giant clouds in which a galaxy or a cluster of galaxies resides within. Dark matter is different from regular matter because regular matter can be detected through electromagnetic waves which dark matter shouldn't produce, unless dark matter self annihilates.

If dark matter is thermal weakly interacting massive particles (WIMPs) then it may produce observable signals when it self annihilates. A popular model for the WIMP which would self annihilate is the neutralino (see figure at right). Self annihilation is exactly what it sounds like, like two identical but opposite forces meeting, the result is an explosion of energy and particles (the interaction conserves energy, momentum, and other quantum numbers). WIMP self annihilations into positrons and electrons could be detected by cosmic ray detectors. These self annihilations and the observable signal would be rare though. If they were common we would see a lot more signal. In the early universe these annihilations would have been much more common such that if there was a primordial abundance of dark matter it would have self annihilated to be consistent with the much lower density of dark matter we observe today (to wrap your head around this take my contrived analogy of a king who has many identical twin sons all born at once, in time they might murder each other until there was enough land for the remaining sons to all have just enough). Mathematically this is stated that the density of dark matter today, Ω, is proportional to the inverse of the particle cross section times relative velocity at freeze-out, σν, (think of this as probability the particles would interact). Today dark matter is said to have frozen out at its current density because on average a dark matter particle will travel the entire distance across the universe (this distance changes also invoking the Hubble scaling
h) before interacting with another dark matter particle:
This is known as the WIMP miracle. Particle physics independently predicts a particle with the right density to be the dark matter that astronomers observe.
Evidence for WIMPs
Recent observations from experiments and collaborations including
ATIC,
Fermi/GLAST,
PAMELA, and others have observed an excess over the expected background of cosmic ray positrons (I am lumping these experiments together, but they actually detect subtly different kinds of particles and found different kinds of anomalies; for example PAMELA detected an upturn in the positron fraction
e+/(
e++
e-) from 10-100 Gev while ATIC detected and excess in the total
e++
e- count at energies of 300-800 Gev). The reality is that anomalies are not unexpected given the uncertainty in astrophysical foregrounds which vary with energy.
Cosmic rays are charged particles (like positrons,
e+,and electrons,
e-) fired like bullets moving close to the speed of light at random throughout the universe and may be created by any of your favorite high energy astrophysical sources like magnetars, super massive black holes, supernovae, and so forth. Cosmic ray particles (any very fast moving charged particles) can interact with the galactic magnetic field, the interstellar medium, or the interstellar radiation field. These interactions generally cause the particles to lose energy and that energy is emitted in the form of observable photons. Thus dark matter will leave an astronomical signature not only in the form of cosmic rays from direct annihilation into positrons or electrons as discussed above, but also in the form of scattering of photons. The reason for all this talk about cosmic rays is that now we can see that the dark matter models explaining the PAMELA, ATIC, and Fermi results would also produce other observables, for example, an excess of gamma ray photons from the galactic center (they would be focused from the galactic center because that is where the dark matter would be densest and annihilating most rapidly) at energies 100 GeV and more. Another signal that may come from dark matter was seen in observations made by the
WMAP satellite which detected residual microwave emission from the galactic center known as the 'WMAP haze'.
You can't just ask a particle where it came from. The electrons and positrons of WIMP annihilation are cosmic rays with an exotic origin, but because there may be an undetected 'local' pulsar producing the cosmic ray anomalies being detected we do not yet know where the positron excess originates. The interpretation of the photon background can be similarly difficult. It is well known that the cosmic x-ray background is the cumulative emission from active galactic nuclei (it can be modeled with a single power-law), but on top of this background local astrophysical phenomena and or dark matter annihilation may contribute to the signal. Astrophysicists are curious to know if dark matter annihilation is necessary or
do cosmic rays account for all the gamma rays in diffuse galactic radiation? The most promising and ongoing source of data to constrain WIMPs comes from the Fermi collaboration. Despite initial excitement in the astrophysics community and the blogosphere about the possible origins of the anomalies (if you don't read the physics blogosphere see
here or
here) the Fermi collaboration now states their results on the measured cosmic-ray spectra are consistent with a standard model of
diffuse galactic gamma-ray emission,
The new result, based on the initial ten months of science observations, significantly improves and extends our knowledge of the isotropic diffuse emission at the high-energy end. The observed emission is softer and lower in intensity than measurements with EGRET had indicated previously. Fermi’s observed spectrum is consistent with a single power-law description over nearly three orders of magnitude in energy
In conclusion the prospect for direct astrophysical observations of dark matter is overwhelmed by uncertainty in the backgrounds and only more careful observations will improve this situation. I should reiterate that the uncertainty is in the detection of dark matter signature, and not the presence of gravitating dark matter.
Making dark matter consistent with observations
Despite the uncertainty in observations we may still draw conclusions and place bounds on possible dark matter candidates. The observed relic density implies that the dark matter annihilation cross section times the relative velocity at freeze-out is very small (see equation above). Unfortunately, given this predicted annihilation cross section the positron signal would be 2 to 3 orders of magnitude smaller than the observed cosmic ray excesses! In order for the WIMP model to fit the various observations a cross section much larger than that predicted by the relic abundance is necessary. Various unknown forces (the dark sector) could increase the annihilation rate. Some researchers have suggested the neutralino WIMP with Sommerfeld enhanced annihilations may explain the observed cosmic ray excesses. Sommerfeld enhancement increases the cross section of particles at low velocities similarly to classical gravitational enchantment. To wrap your head around this imagine dark matter particles as strangers quickly passing in the street; dark matter particles and strangers don't bother to hang out with each other at this speed, but if they stop at a coffee shop then the possibility that strangers would have some kind of interaction is greatly enhanced. If dark matter particles are jetting around too fast they wont have any interactions, however, if the particles slow down a significant interaction may occur between them. The classical gravitational enhancement analogy can quantify Sommerfeld enhancement a little more. Consider a particle encountering a star of radius R and mass M so the cross section should just be πR2 in the case that the particle directly hits the star, but as the particle approaches the star at a velocity ν it is trivial to show using conservation of energy and angular momentum that the cross section is increased to
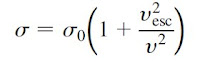
where ν
esc2=2GM/R. The Sommerfeld enhancement is the quantum extension of this classical phenomena. If you work through the entire derivation the result is that the enhacement leads to a cross section that scales at low energies as σν ∝ 1/ν. It turns out that the velocity of dark matter particles is a factor of ~10
- 3 less now compared to what it was at freeze out. It is a miracle of dark matter that Sommerfeld enhancement provides an elegant mechanism for boosting annihilations now to exactly the amount needed.
However, a satisfactory solution to the dark matter problem must not only have dark matter annihilating at the correct rate, but it must also produce the right density and structure on all cosmological scales which is consistent with observations. In a paper appearing in Physical Review Letters, Jonathan L. Feng, Manoj Kaplinghat, and Hai-Bo Yu of the University of California, Irvine, point out some problems with these explanations, in thier own words from the abstract:
Dark matter with Sommerfeld enhanced annihilation has been proposed to explain observed cosmic ray positron excesses in the 10 GeV to TeV energy range. We show that the required enhancement implies thermal relic densities that are too small to be all of dark matter. We also show that the dark matter is sufficiently self-interacting that observations of elliptical galactic dark matter halos exclude large Sommerfeld enhancement for light force carriers. Resonant Sommerfeld enhancement does not modify these conclusions, and the astrophysical boosts required to resolve these discrepancies are disfavored, especially when significant self-interactions suppress halo substructure.
The authors find that the relic density cannot be achieved in any Sommerfeld enhanced interaction scenario even with additional resonant boosting terms. What is more, they find that the Sommerfeld enhancement would create significant changes in dark matter halos because the additional interactions would isotropize the velocity dispersion and create spherical halos that contradict observations of NGC 720. The effects of dark matter self interactions can be seen in almost any x-ray image of a galaxy or cluster galaxies. For example, the image above of the galaxy cluster
CL0025+1654 shows the dark matter (shown in blue) has an approximately thermal distribution and an elliptical shape. Subhalos with high density are also predicted to be present (in the image above the subhalos are not resolved, but many N-body simulations have predicted them to be there) with lower velocity dispersions than the thermal bulk of the halo, such that the Sommerfeld enhanced cross sections make these components especially important. Further observations of halos and subhalos will place even more constraints on dark matter self interactions.
I must comment on the authors findings on spherical halos. I would agree that enhanced interactions can create significant changes in the shapes of dark matter halos, but I caution that analysis of a single galaxy is not sufficient to constrain dark matter. Observations of our own Milky Way indicate that our dark matter halo probably looks like a
beach ball flattened perpendicular to the visible plane of the galaxy. This would corroborate the authors findings, however, I have had discussions with colleagues on dark matter forming spherical halos. Finally, it should be obvious that any dark matter model that calls for heavily interacting dark matter would produce wildly inconsistent results. One theory that is been proposed is that there is a hidden world of mirror-matter that acts just like regular matter, but it doesn't interact with regular matter through any force but gravity. Mirror galaxies, mirror stars, and mirror planets are completely inconsistent with observations! The experiments discussed above placed constraints on the type and mass of dark matter particles that would rule out such mirror-matter. I did not include or discuss the
DAMA experiment's observations earlier because they had not been reproduced independently by any other group until very recently. The
CoGeNT experiment now has a confirmation of the DAMA signal and this may change things by allowing the considered masses of dark matter candidates to be reduced considerably (this still doesn't allow for mirror-matter although some are implying it does). I refuse to equivocate my opinion that there is no hidden sector which is an exact copy of the standard model.
A survey of the lessons and knowns about dark matter indicates that we need more data. Dark matter models motivated from cosmic positron observations require an enhanced cross section to reproduce the data, but this enhanced cross section is inconsistent with the relic dark matter density and observations of dark mater halos. We need observers to determine if the cosmic positron excess is caused by a local astrophysical source such as a pulsar. We need theorists to develop a simple model for dark matter that is motivated from fundamental particle physics that make testable predictions for future observations.

References:
Abdo, A., et al. (2010). Spectrum of the Isotropic Diffuse Gamma-Ray Emission Derived from First-Year Fermi Large Area Telescope Data Physical Review Letters, 104 (10) DOI: 10.1103/PhysRevLett.104.101101
Nima Arkani-Hamed, Douglas P. Finkbeiner, Tracy R. Slatyer, & Neal Weiner (2008). A Theory of Dark Matter Phys.Rev.D79:015014,2009 arXiv: 0810.0713v3
Feng, J., Kaplinghat, M., & Yu, H. (2010). Halo-Shape and Relic-Density Exclusions of Sommerfeld-Enhanced Dark Matter Explanations of Cosmic Ray Excesses Physical Review Letters, 104 (15) DOI: 10.1103/PhysRevLett.104.151301